February 3, 2021
Navigating Psyche
I’m Drew Ryan Jones, a member of the mission design and navigation (MDNAV) team tasked with charting and navigating a course to the asteroid (16) Psyche. A general description of the Psyche mission is found at https://psyche.asu.edu/.
As a child I was fascinated with maps (the paper kind), atlases, and globes. I would look for optimal or complex routes between interesting places and digest the statistical information contained within. Planets and stars in the sky appeared like places on a map and I wondered what the roads could look like. Now, as an adult, I use math and computer programs to help discover and assess those routes in the sky. Generally speaking, I am a spacecraft navigator. My job involves designing, refining, and assessing the accuracy of trajectories and orbits for interplanetary spacecraft.
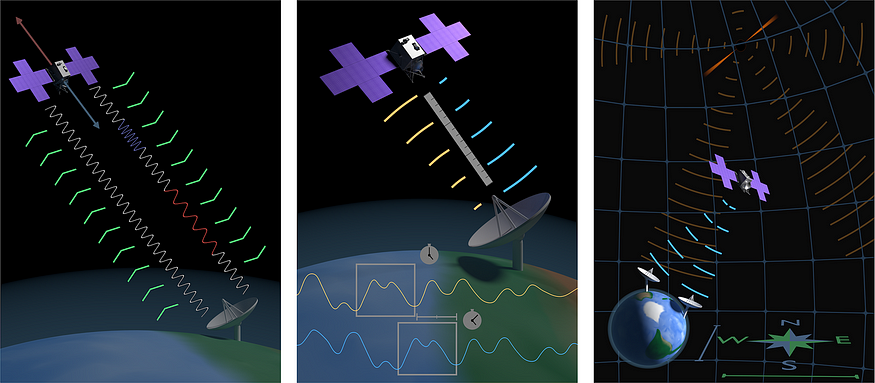
“Navigation Data Types Triptych”, digital media, by Ben Conway
So how exactly are we designing the trajectory from Earth to our destination of Psyche? What does Mars have to do with anything? And what are the unique navigational challenges for this mission compared to other robotic missions? In this article I will attempt some answers. This will be a “30,000-foot-view” of the Psyche mission trajectory and navigation. Or perhaps a “3.3-au-view” (that being the furthest distance from the Sun our spacecraft will reach). This article will focus on navigating from Earth to Psyche. The topic of how we precisely navigate our spacecraft about a largely unknown body is left for another time.
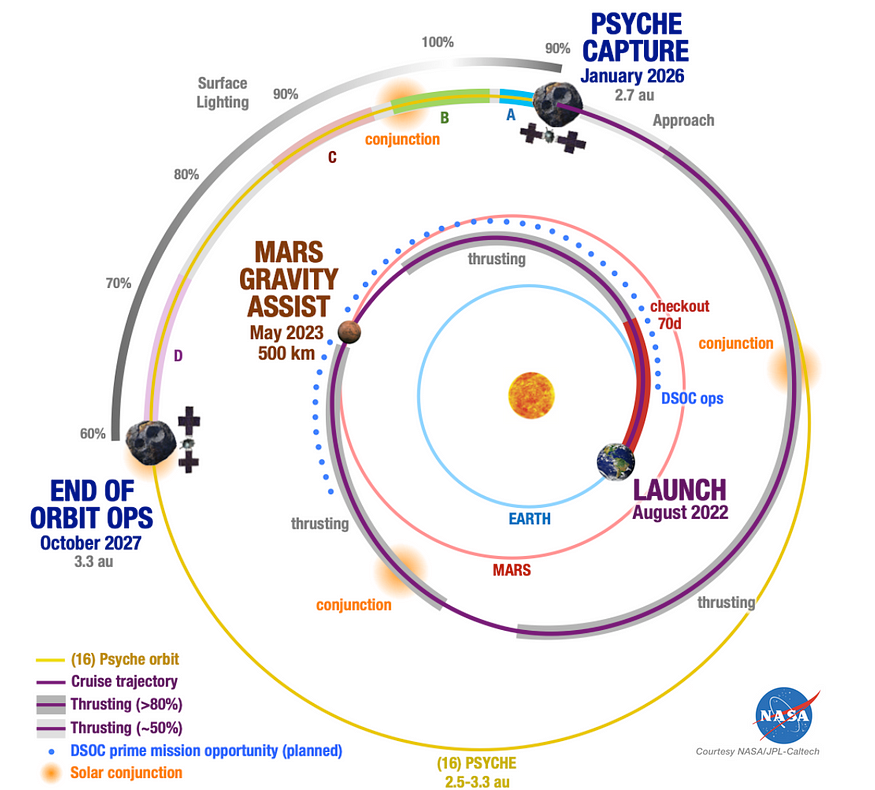
The Psyche Mission Overview
Like all subsystem teams, MDNAV must work to ensure we satisfy requirements levied on us by other teams. The primary requirements for the overall trajectory design is to meet the science goals. We also have constraints on spacecraft mass, power, fuel, and location accuracy. The use of electric propulsion makes this project unique in many ways. Electric propulsion has been discussed by some of my colleagues here and here. Many space missions rely on higher thrust chemical propulsion and a designed route which remains relatively fixed after launch. But with electric propulsion we will be frequently adjusting our course and re-designing our path to Psyche. The adjustments account for variations in the performance of the electric engine and other uncertain dynamics. The SpaceX Falcon Heavy launch vehicle sends our intrepid explorer with speed to escape the gravity of Earth and in the general direction of our mysterious target asteroid. But we can’t get the full mass of the vehicle there without a little help from some friends. In this case, our electric propulsion system and the planet Mars. The propulsion will operate for most of the mission, but even if we operated the engine the entire time we cannot meet our requirements without the boost provided by flying close to Mars. Technically, our vehicle will exchange momentum with Mars. A bit like a skateboarder grabbing on to the back of a turning truck, Mars’s gravity will change our spacecraft’s speed and direction, swinging us outward. This is known as a gravity assist and it is a critical element of the mission.
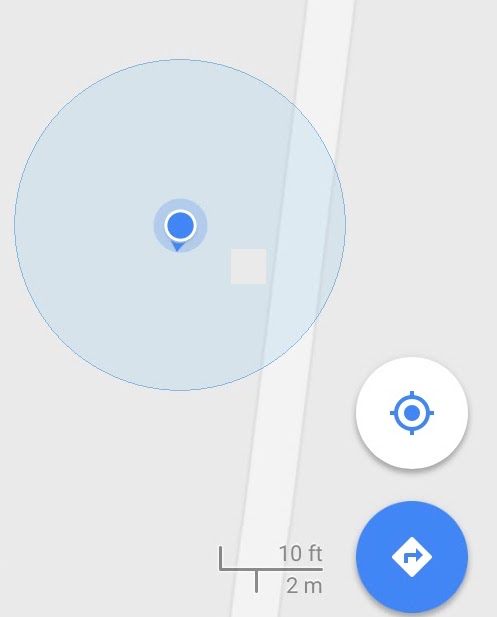
The GPS navigation software on your phone indicates the region of uncertainty in your location
When navigating spacecraft we never precisely know the location of our vehicle, rather we can only determine the location within some bubble of uncertainty. It comes down to probability. We determine with some certainty, like 99%, that the vehicle is within a region of space. It is analogous to the position circle you see around your location when using your phone’s GPS navigation.
So how precisely will we know our spacecraft’s position? In order to properly point the deep-space optical communications (DSOC) experimental laser we need to know the position to within 0.5 millidegrees before encountering Mars and down to 0.08 millidegrees between Mars and Psyche. This accuracy is equivalent to aiming from Los Angeles to New York City and hitting a 1-meter target! We achieve this knowledge by taking measurements via a radio signal sent between our spacecraft and tracking stations on the Earth known as the Deep Space Network or DSN. We precisely measure the time it takes for this signal to travel at the speed of light, and this provides a measurement of the distance or range. We also measure how the signal is frequency shifted which provides a measure of spacecraft speed. The frequency shift is analogous to how a siren from an emergency vehicle has a higher or lower pitch depending on whether it is is approaching or receding from the listener. These measurements are not perfect and are subject to various sources of error, such as delays when the signal passes through the atmosphere. We model all relevant effects and adjust our models to best
fit the data. Later in the mission we will also use pictures of the asteroid’s surface to enable our orbital operations. With enough data and sufficiently good models we can achieve our accuracy requirements.

Fictitious spacecraft uncertainty ellipses in two dimensions during a flyby of Mars
There is much work to be done prior to launch to ensure we can execute the mission and that we are robust to various errors. Through detailed analyses we have demonstrated that we can get to Psyche even if we lose some thrusting due to unplanned spacecraft problems. We also determine how much DSN tracking we require and how often we need to update the spacecraft with knowledge of where it is located. After launch we execute this plan but will also need to adjust to the expected unexpectedness of the space environment.
I am truly grateful to be working as a modern explorer, adding depth to the maps of our solar system. This mission is a massive team effort, and I feel very lucky to be on such an exceptional team and a team led by my alma mater…Go Sun Devils! We are prepared to navigate the challenges ahead. Our excitement for launch grows greater every day!
Drew Ryan Jones: Spacecraft navigation engineer for “Psyche: Journey to a Metal World” at NASA’s Jet Propulsion Laboratory, California Institute of Technology